The Sycamore Quantum Entanglement Experiment
Probing the implications of ER = EPR theory. (Wormholes = Quantum Entanglement)
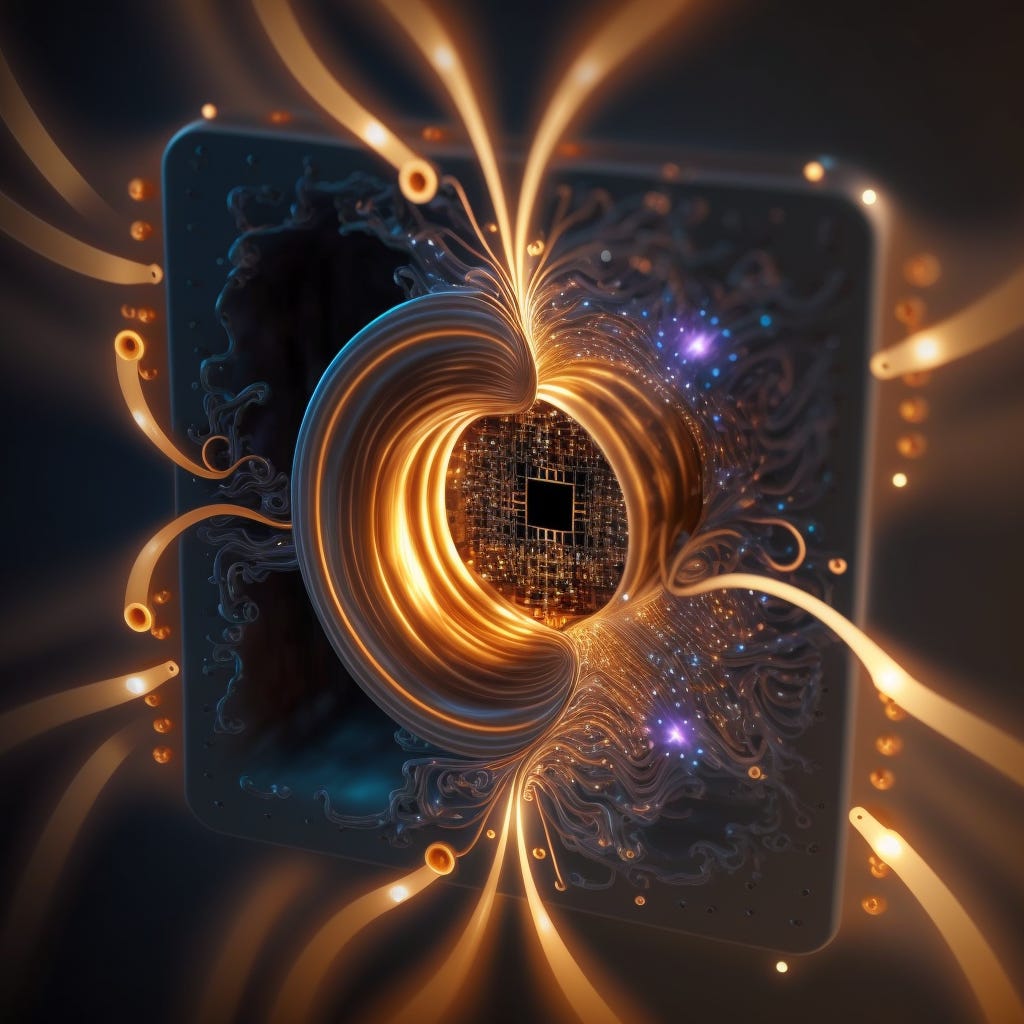
The recent quantum experiment carried out on Google’s Sycamore quantum computer could have profound implications for physics. In the media’s effort to craft breathless headlines and invoke images of wormholes from Star Trek or Marvel movies, they buried what could end up being an important moment in our understanding of the universe.
The experiment didn’t create a wormhole in the way we think of wormholes in fiction. If we’re trying to be precise, we should say they used a quantum computer to create a very specific system of entangled particles to test some intriguing properties. The properties they tested are theoretically consistent with a traversable science fiction style wormhole but only in a very specialized theoretical framework. It has lots of implications for quantum computing and information transmission, but we’re nowhere near talking about portals to other parts of the universe.
They are however, tugging at the threads that tie up two paradoxes in physics, namely, quantum entanglement and the black hole information paradox. By linking these two paradoxes, physicists may have found a way to resolve both paradoxes with each other. Entangled particles may be connected by wormholes. Maybe. What they’ve found is enough to send a tingle across my shoulders.
To understand all this, we need to take a walk through the park of mind-bending physics. It’s a fun hike, but we need to watch where we step.
Quantum Entanglement
First up, let’s talk about quantum entanglement. You’ll remember from high school chemistry that electrons occupy orbitals in pairs. They either have spin up or spin down, but due to the Pauli Exclusion principle, electrons must have opposite spins in a pair. We say that pair is entangled. If one electron changes spin, the other must change as well, instantly. Their spin fates are bound. The same principle applies to other particles, but let’s keep it simple and just worry about our two electrons.
Imagine that you separate these electrons by great distance and keep them in a highly controlled environment, preserving the spin of each. You take the spin up electron by rocket to the moon and keep the spin down electron on Earth. You record simultaneous, synchronized measurements of each electron, every tenth of a second, measuring its spin.
Now, imagine you flip your spin down electron on Earth to spin up. The measurements of the electron on the moon would show it instantly flips the opposite direction to obey entanglement. Fair enough.
But the moon is 1.3 seconds away, and the change occurred within a tenth of a second. How could the information from one electron travel to the other faster than the speed of light? Nothing can go faster than the speed of light. The laws of physics are absolute on this, fundamental to both general relativity and quantum mechanics. So what gives? How did the electrons know to swap spins faster than the speed of light? That demonstrates the paradox of quantum entanglement.
The Black Hole Information Paradox
Black holes, as described by general relativity, are shockingly simple, elegant objects. All you need to describe them mathematically is their mass, their spin, and their electric charge. Three numbers. That’s it. Jot them down and go home. That’s all we need to describe your favorite black hole. That’s all we’ll ever know about it because that’s all we can ever know about it.
We learned in grade school that nothing escapes a black hole, not even light. Anything that falls into a black hole’s unrelenting gravitational grip is lost forever. The mass, energy, and unfortunate daredevil aliens that got too close are churned into the mass-energy of the black hole and become a part of it. Photons, protons, neutron stars, along with anything else you’d like to throw in there, is lost.
That means the information about things falling into a black hole, the mathematical description of their status and the characteristics of their constituent particle interactions, is also lost. That creates the black hole information paradox for quantum mechanics. According to the laws of quantum mechanics, quantum characteristics are conserved. Information is conserved.
That isn’t to say you can’t change the form of information. Introduce some plasma to light fire to a book and you’ll wreck the book, but the information about the state of the matter and all its bits is still there in a charred pile or it fled throughout the room in the form of photons or smoke. You could, if you had sufficient technology to observe all the book particles (and more patience than God), presumably reverse the burning of the book and reassemble it atom by atom and photon by photon. Or maybe, imagine Doctor Strange shows up, gives the time stone a twist and runs back the universal clock. All the atoms and particles are still there, just moved around, so you see them all return to their original seating arrangement in the book. The point is, burning the book is theoretically reversible, if practically impossible. But no matter how hard you try, once you throw the same book into a black hole, the information is truly lost, according to general relativity. The process is irreversible.
In contrast to the general relativity, black holes, according to quantum mechanics, are incredibly complicated, containing the information of everything within the black hole.
This gets even weirder, but stay with me.
If black holes obey the basic laws of physics, they must also have entropy. Stephen Hawking tried to prove that they didn’t have entropy. He ended up famously proving that they do indeed have entropy and that they will radiate particles away, very, very slowly through what we now call Hawking Radiation. Yes, give them a long enough time and not enough fuel to consume, and black holes will evaporate back into the void.
That makes the information paradox even more important, because we could now have particles that were once a part of black hole roaming the cosmos, and, according to general relativity, we’d have no idea where they came from. The black hole information paradox is no longer limited to black holes.
ER = EPR
These two paradoxes may be resolved, using each other by an elegant theory demonstrating quantum gravity, known as ER = EPR.
Einstein published two papers in 1935, one with Nathan Rosen and another with Rosen and Boris Podolsky, hence, ER for one and EPR for the other. Einstein hated the paradox of quantum entanglement, famously dubbing it “spooky action at a distance.” Surely, what quantum physicists had come up with was wrong, somehow, somewhere, to generate such a weird impossibility.
To demonstrate how ridiculous entanglement was, he devised a thought experiment with Podolsky and Rosen. This EPR paper had the opposite effect, however, eventually lending credibility to the paradox. For that reason, many historians think Einstein actually helped show entanglement was a real gap in our understanding of the universe.
Einstein also wrote a less well-known paper with Rosen, the ER paper, demonstrating that black holes could come in pairs, connected by shortcuts through space. These are so-called Einstein-Rosen bridges, what we commonly call wormholes. Wormholes have, as you know if you like modern science fiction, are a common trope in stories from Contact to Thor. Anytime you’re watching a movie and you see a scientist character jam a pencil through a piece of folded paper (seriously, Hollywood only has the one analogy for this) to explain the faster-than-light or interdimensional travel that’s about to happen, they’re talking about an Einstein-Rosen Bridge.
In 2013, scientists realized that quantum entanglement, EPR, could be described by wormholes, ER. The revelation that ER = EPR was a stroke of mathematical genius, if not one that describes the physical world. At the time, the idea was considered a fringe notion worth investigating. In the years since, evidence has mounted. In 2017, Harvard University's Daniel Jafferis expanded the ER = EPR theory to traversable wormholes. ER wormholes would be inherently unstable and not useful the way science fiction depicts, but a potentially traversable wormhole, stabilized by properties inherent to entanglement, opens a universe of possibilities, maybe not practical for sending yourself through, but maybe for sending particles through, transferring information across space via entanglement.
In 2015, another team showed that a simplified quantum system's properties could be equivalent to quantum gravity effects. That meant that it might be possible to actually test ER = EPR on quantum processors. Whenever a theory like this becomes testable with experiment, it suddenly draws more attention. ER = EPR entered the mainstream, and now we’re cooking.
The Holographic Principle
Back to black holes a moment. Another theory is that no information is lost to black holes because it’s stored on the surface of the black hole through something called the holographic principle. Now, before you let your brain run away with ideas of holograms and the universe being a simulation, remember that physicists constantly conflate terminology with vaguely related things in the practical world. The holographic principle here simply explains mathematically how information might be stored on, or in, a black hole. It relates to how we can mathematically describe spacetime. Physicists will debate endlessly if black holes even have “an inside” in the way we casually think of it, or if their surface, the event horizon, represents a three-dimensional limit of space where the laws of physics hold true. But like I said, let’s not get tangled in the weeds here. The point is, there’s a theory of how information could be preserved in, or on, a black hole.
By applying two different descriptions of the structure of spacetime to the holographic principle, a duality arises, meaning that in certain circumstances, those two descriptions resolve to the same thing. This turns out to be another key to understanding the potential relevance of the experiment on the Sycamore chip. The experiment didn’t create a wormhole per se, but it did, perhaps, show that entangled particles in normal vanilla spacetime can also be described as wormhole between the particles.
The Sycamore Experiment
In the interest of time and space (pun very much intended), I’m going to oversimplify my description of the experiment here, but the basic model is that they set up two pathways. The first was a control that moved qubits (quantum bits of information) through a more conventional pathway. The second was the experimental course that “pushed” qubits through qubit-sized wormholes. At the end of both pathways, they measured the amount of entanglement on each set of qubits. The qubits sent through the wormhole path maintained more entanglement indicating that information had, indeed, been transmitted via a mechanism similar to what they’d expect if it went through a traversable wormhole.
One of the reasons that traversable, human-sized wormholes remain firmly in the real of science fiction is that they would require an enormous amount of energy and an enormous amount of negative energy. Negative energy isn’t possible in classical physics, but it’s everywhere in quantum physics, notably arising from vacuum energy that permeates all space and causes virtual particle pairs to pop into existence and immediately annihilate each other. With a “push” or “shock” of negative energy, they prop open these quantum shortcuts. Wormholes at the macro scale are impossible but may be common at the quantum scale.
What Does it All Mean?
Paradoxes Resolved?
We can almost certainly assume that Einstein, Rosen, and Podolsky would have loved this experiment because it shows that, at least in a very specific, simplified case, the entanglement paradox can be explained by wormholes. The paradox is avoided because information doesn’t travel faster than light. Instead, it takes a shortcut through a wormhole. It also provides one possible way to avoid the black hole information paradox. Entangled particles in a black hole may be connected by these sorts of quantum wormholes.
The Big and Small Picture
Broadly, if ER = EPR is correct, it shows us that quantum entanglement and wormholes aren’t disconnected paradoxes in different fields of physics. They’re two manifestations of the same underlying principle. In that scenario, unification of ER and EPR could form the foundation of all spacetime. Quantum entanglement creates the structure or shape of space, and without entanglement, space as we know it would not exist. And, if general relativity and quantum mechanics can be connected in these circumstances, ER = EPR might give scientists the mathematical foothold for unifying gravity with quantum mechanics.
For me, experiments and theories like ER = EPR compel us to explore intriguing questions. The closer we get to probing quantum gravity and known paradoxes, the closer we should eventually get to filling big holes in our understanding. From an astrophysical perspective, I’m intrigued that entangled particles could explain the shape or structure of spacetime. If the fundamental pieces of the universe behave like waves, preserving information as they go, what is waving? Is it the fabric of spacetime? But what is even “the fabric of spacetime?” Is the universe only the sum of its quantized parts, a foaming three-dimensional sea of particles and the interactions between them? Or is there something else, like an underlying geometry of the universe—the shape of the universe—that also determines the smallest possible scale of fundamental particles.
If space itself is only the result of what’s in space, with its behavior, structure, and dimension is determined by only what can be interacted with through one of the fundamental forces? Is it all just matter particles and force-carrying particles interacting to fill what we consider the void?
If space is only the result of particles and interactions between particles, some of which are entangled and connected by wormholes, what does that mean for relative measurements of basic quantities like length, mass, and time?
Okay, But What Does it Really Mean?
Practically speaking, this is not leading us to futuristic wormhole space travel. Some scientists believe that it might give us some theoretical insight into using quantum entanglement to prop open and stabilize Einstein-Rosen bridges, but applications to anything beyond the quantum scale are speculative, at best.
Regardless, probing ER = EPR is leading us to ways to store, process, and send information faster. That alone will have major implications for future technologies. Remember that the electron was discovered only in 1897. Less than a century later, our world was fundamentally transformed by electronics. Chasing fundamental questions leads us places we didn’t know existed. Likewise, no one knows for sure where ER = EPR will take us, but it’s certainly worth exploring.